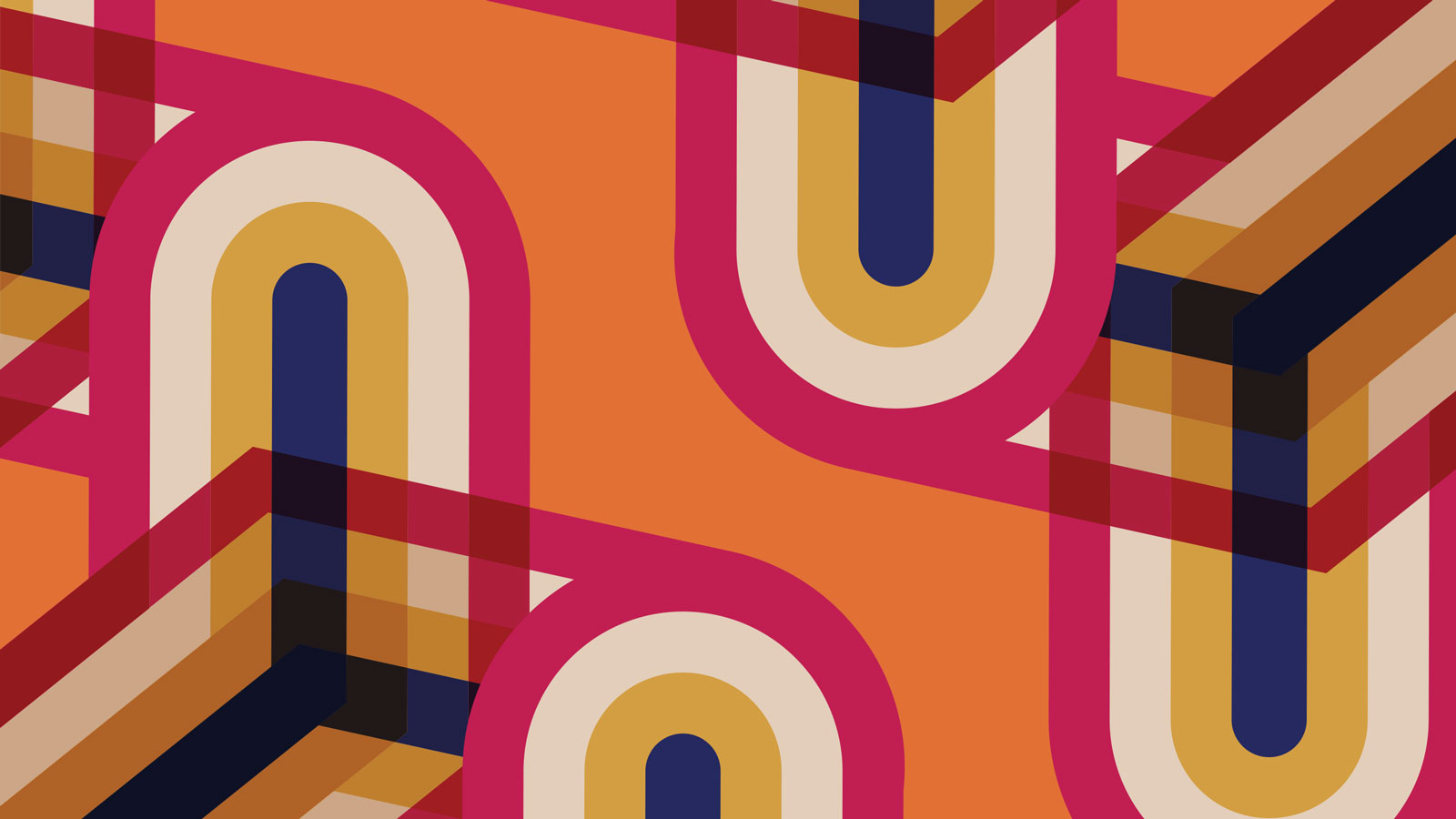
In this article, we examine the different ways cancer escalates the conflicts within our bodies, and how scientists are leveraging the incredible power of genetics and genomics to disarm it.
For most of human history, cancer was considered a monolithic enemy — a mass that grew and eventually spread to vital organs such as the lungs or brain, eventually killing the patient. Weapons of mass destruction were directed against this enemy: surgery (accounts go back to antiquity), radiation since the early 1900s, and chemotherapy since around 1940. Some patients shorthand these unpleasant options as “slash, burn and poison.”
The so-called war on cancer, initiated with the National Cancer Act of 1971, didn’t wipe out cancer, but it did launch an era of much better profiling of the enemy. Cancer, it turned out, is not one disease but many, and cancers are best characterized not by location in the body but by mechanisms (such as the close links between breast and ovarian cancers).
Today’s search for cancer cures is more closely related to hunting down cyber-criminals than conducting a pitched battle in a theater of war. Just as cyber-criminals use tricks of digital code to defraud and delude their victims, cancers manipulate genetic code to wreak havoc on the body through a variety of mechanisms. These include hijacking the body’s immune system, activating invasion and metastasis, and turning off normal growth suppressors and cell death.
Every cancer involves genome instability — tiny errors in genetic code that accumulate and turn normal cells into destructive invaders. The scientists of the JAX National Cancer Institute-funded The JAX Cancer CenterThe Jackson Laboratory Cancer Center (JAXCC) is a National Cancer Institute-designated Cancer Center complemented by institutional education, resource and service initiatives that support cancer research world-wide. The JAXCC comprises approximately 50 members with multidisciplinary expertise who are united in research aimed at understanding and targeting the genomic complexity of cancer.Cancer Center focus on decoding and disarming cancer’s genomic changes, with the goals of finding cancers before they cause damage and neutralizing them with targeted treatments.
Read on for five ways JAX scientists are winning the cancer arms race.
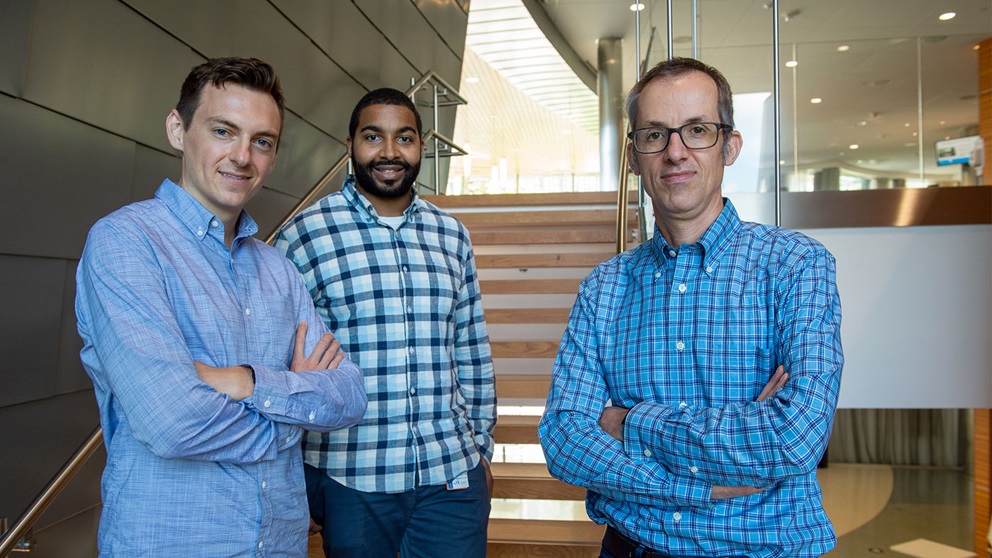
JAX Professor Roel Verhaak (right) and members of his cancer research team at JAX Genomic Medicine in Connecticut. Photo by Cloe Poisson.
1. Finding the cancer dangers that are hidden in plain sight
In the hunt for genetic cancer risk factors, researchers painstakingly analyze huge amounts of genome sequence data. They look for specific mutations that increase or decrease susceptibility, as well as larger-scale genomic patterns that may do the same.
Research has led to tests that identify the mutations driving cancer in individual patients as well as treatments that target those specific cancers.
But all along, there have been cancer‑causing genetic anomalies that remained hidden from standard sequencing protocols. One kind is found within the genome itself: structural variants (SVs), including duplications, insertions, deletions and inversions of normal DNA sequences, that are difficult to spot because they don’t change the sequence data. Recent advances in long‑read sequencing are revealing how common SVs are even in healthy people, and they have also been implicated in multiple cancer types.
Now the significance of another cancer danger is coming to light: extra-chromosomal DNA (ecDNA). This DNA somehow becomes separated from the chromosomes themselves and forms a circular structure in the nucleus. Its segments may contain many copies of various genes, adding to the two copies found, as usual, in the chromosomes themselves. And if ecDNA sequences contain cancer-promoting genes, it can cause havoc.
Cancer initiation
Advanced cancers often have severely disrupted genomes, but the mutation or dysregulation of just a single gene can start cells on the path to malignancy.
A variety of factors can cause cancer-related genes to produce either too much or not enough of a protein. Loss-of-function mutations or deletions of genes such as BRCA1 and BRCA2, which promote the regulation of cell division and DNA repair, and TP53, a tumor suppressor also involved with suppressing cell proliferation, are well-known as cancer risk factors. Other genes, known as oncogenes, become dangerous when they are over-expressed. Oncogenes such as EFGR and Ras produce proteins that promote cell growth, differentiation and proliferation, while myc regulates the expression of many such proliferation-related genes.
When a DNA segment breaks away to form ecDNA, it loses the usual systems that control gene expression. Therefore, ecDNA can be highly transcribed and, if it contains any coding genes, yield an abnormally large amount of protein. When those genes include oncogenes, the normal checks and balances on cell growth and division can be overwhelmed by the uncontrolled protein production, leading to cancer initiation and tumor formation.
Implicated in cancer
It has become increasingly recognized that genomic lesions, which increase oncogene expression, play a role in some cancers, including SVs within chromosomes and ecDNA formation. Researchers first spotted ecDNA in cancer cells decades ago in quite a literal fashion, using fluorescent probes that bound to specific sequences and lit them up, showing that they were physically separate from the typical chromosomal structures in the nucleus. There were still questions surrounding both the prevalence of ecDNA in cancer and the role(s) it might play in disease progression and treatment strategy.
JAX Professor has been investigating aspects of ecDNA, particularly in the context of glioblastoma, a brain cancer that is highly resistant to conventional cancer therapies. He found that ecDNA is unevenly inherited by daughter cells during cancer cell divisions, creating important differences between cell populations. It makes sense — while chromosomes are evenly divided in a highly controlled process during cell division, ecDNA has no such regulation and is passed to daughter cells seemingly at random. The differences increase as the cells continue to divide, creating what is known as genomic heterogeneity within the tumor. If there are many sub-populations of cells with different properties, it becomes very difficult to find an effective therapy strategy to eliminate all of them. Therefore, ecDNA not only contributes to glioblastoma initiation, it can also provide a mechanism for therapy resistance and tumor recurrence.
More recently, Verhaak and colleagues took a broader view to assess ecDNA frequency and clinical impact across multiple cancer types in thousands of patients. They found that ecDNA is far more common in a variety of cancers than previously thought — by more than 15-fold— and while its presence is often amplified in cancer cells, it is not in normal tissues. Furthermore, patients whose cancers carry ecDNA with increased oncogene expression have more aggressive cancers than those without. Indeed, ecDNA amplification in tumors was associated with significantly worse five-year survival outcomes.
Is ecDNA a key to stopping therapy-resistant cancers?
The discoveries are a crucial first step toward finding preventions or effective therapies for these dangerous cancers. The insight into ecDNA’s prevalence and importance will fuel exploration into potential clinical targets. For example, how does ecDNA form? Can it somehow be neutralized before it can cause harm? Also, how can diagnosing ecDNA-positive cancers help guide treatment strategies? The findings that ecDNA may underlie some of the most difficult-to-treat cancers indicate that new therapeutic approaches are needed to improve patient prognoses.
While learning that ecDNA increases cancer lethality may be alarming, knowledge is power.
“We are on a mission to improve the outcomes of patients with cancer,” says Verhaak, “and our ecDNA discoveries are pivotal for achieving those goals.”
JAX scientist Gary Ren (left) and colleagues at his Maine-based cancer research laboratory. JAX photo by Tiffany Laufer.
2. Derailing cancer metastasis
'Metastatic cancer is the most dangerous and remains the most lethal.'
As cancer therapies improve and grow ever more precise, many cancers can be eradicated or effectively shut down at their site of origin. While medically serious, these primary tumors usually have growth pathways that are targetable, and there are now many effective treatments available. Nonetheless, there were 9.5 million cancer deaths worldwide in 2018, and that number is expected to jump to 16.4 million by 2040. So why do so many patients still die?
Unfortunately, not all cancer cells are the same, even within the tumor, and not all of them stay put. Some break away from the primary tumor and move to other locations in the body, in a process known as metastasis. Metastatic cancer is the most dangerous and remains the most lethal. Understanding the processes and variables underlying metastatic cancer and how it might be stopped is therefore essential if we are to make the next leap forward in improving cancer care.
How does cancer spread?
When cancer begins, it usually involves a single rogue cell. Somehow the brakes come off the carefully controlled cell division cycle, allowing the cell, and eventually its many descendants, to grow and divide with relentless speed. As it grows, it quickly adapts to and co-opts biological systems within its particular organ or tissue.
At a certain point, however, cancer cells begin to move, either by growing into other tissues or separating from the original tumor. Cells that break away travel through the blood stream and lymph system to other places in the body. These cells face long odds, and most of them are eliminated by the immune system or fail to adapt to the environment in which they settle. Sometimes they escape immune surveillance for long enough, and adapt to their new environment quickly enough, to survive the transition. Eventually—weeks, months or even years later—they begin to thrive once again and grow aggressively, resistant to treatments that may have worked well for the primary tumor.
Cancer researchers are investigating the steps in the metastatic process to look for ways to stop cancer from spreading. Could immune surveillance be enhanced to eliminate cells before they reach other areas of the body? Could the environments in other tissues be made less hospitable to the cells that infiltrate them? And if they’re not eliminated before reaching a destination, is there a way to maintain metastatic cells in a senescent state, keeping them inactive by undermining their ability to grow and divide? If the answer is yes, and a therapy can be developed that prevents metastatic cancer growth, it would save many lives.
Immune cell roles
Assistant Professor Guangwen "Gary" Ren, Ph.D.To study tumor microenvironment and tumor immunology in cancer therapeutic resistance and metastatic relapseGuangwen “Gary” Ren investigates cancer micro-environments, the normal tissues and cells immediately adjacent to cancer cells and tumors. There are many interactions between cancer cells and their immediate cellular neighbors, some of which are quite important for cancer growth.It’s particularly imperative for the cancer cells to evade and eventually co-opt the local immune cells that might otherwise recognize and destroy them. Not many accomplish this feat, but those that do are able to grow while actually being protected by the immune cells that would normally kill them.
When metastatic cancer cells migrate elsewhere in the body, they’re on their own, and have to deal with a new environment and immune cells. So how are they able to succeed in there, so to speak? Ren is investigating the complex interplay between immune cells and cancer cells, and between different kinds of immune cells, that can dictate success or failure of metastatic cancer spread. Of particular interest are cells known as neutrophils, which are part of the innate immune system, the first line of defense against invading pathogens. Recent research in Ren’s laboratory has shown that neutrophils also play surprising roles in cancer metastasis.
Neutrophil paradoxes
Ren and his team worked with specialized mouse models to look at breast cancer cells that migrate to lung tissue, a common site for breast cancer metastatic spread. They are often eliminated by either neutrophils or natural killer (NK) cells, another component of the innate immune system. In an unexpected result, however, Ren found that when both neutrophils and NK cells are present in lung tissue, the neutrophils don’t react against the cancer cells. Instead, they actually inhibit NK activity, helping the metastatic cells survive and increasing the chance of cancer spread. The finding helps to explain why treatments that increase neutrophil counts in cancer patients, which are often greatly reduced by chemotherapies, are associated with higher risk for subsequent metastatic disease.
In addition, the research team discovered that neutrophils in the lung tend to stock up on fuel in the form of lipids when a breast cancer tumor is growing. Interestingly, the process is stimulated by molecular signals sent from the tumor itself, even before its cells begin to migrate elsewhere. Then, when circulating cancer cells do arrive in the lung, the neutrophils transfer the lipid fuel to them, increasing their ability to survive and proliferate.
Targets for treatment
More research is needed into exactly why neutrophils function as both safeguards against metastasis and, in different contexts, as part of the support system for it. Nonetheless, Ren’s findings provide intriguing targets for therapy refinement and development. For example, assessing NK/neutrophil populations can help inform whether or not to increase neutrophil counts following initial therapy. And disrupting the signaling/metabolic cascade that helps fuel metastatic cancer cells in the lung could greatly reduce the chances for successful spread. These and other treatment regimens are both parts of the larger effort to derail cancer spread and, ultimately, deaths from metastatic cancer.
John Pierce is a cancer patient who is participating in the Maine Cancer Genomics Initiative. Pierce was able to take part in the MCGI tumor board meeting online in March. Photo by Alexandra Giardino.
3. Creating a model for personalized cancer care
From cancer genomic testing to clinical trials, John Pierce is exploring the latest advances to address his medical issues.
What advice would John Pierce give to someone who has just received a cancer diagnosis?
"First, be your own best advocate. When I was a 20-year-old combat helicopter pilot in Vietnam, I learned that when someone or something is trying to kill you every day, you recognize that nothing focuses your mind like your own mortality."
Second, he says, "get genomic profiling."
Nearly 50 years after his combat experience, Pierce is now retired from a successful and varied career as an internet consultant. And following a series of unusual health crises that trained him to seek out the best medical advice and treatment, he has been diagnosed with cholangiocarcinoma — a very rare cancer of the bile ducts, the slender tubes that carry the digestive fluid bile through the liver. Pierce is now bringing his lifelong facility for quickly acquiring technical expertise to his treatment regime, to be his own best advocate.
Pierce may not be your average cancer patient, but every patient, and every cancer, is genomically unique. Pierce says that when he learned he had cancer, “my first inclination was to obtain genomic testing. Cancer isn’t liver cancer or lung cancer; it’s defined by whatever the cancer’s genomic profile is. And that’s why I started down this path and requested testing.”
Pierce’s oncologist is Dr. Roger Inhorn of MaineHealth, a steering committee member of the Maine Cancer Genomics Initiative (MCGI). JAX founded MCGI in 2016 with a grant from the Harold Alfond™ Foundation and has enrolled every oncology practice in Maine and most of its oncologists. In phase one of the program, which concluded at the end of 2020, participating oncologists submitted patient tumor samples to be sequenced and profiled for genes known to be associated with various cancers, and with response or resistance to approved targeted therapies or new drugs in development approved by the U.S. Food and Drug Administration.
“For almost all of my patients who have an advanced malignancy and have enough tissue available,” Inhorn says, “I offered participation in MCGI. Genomic profile testing helps clarify potential options by looking for targetable mutations for which there might be either a clinical trial option or a commercially available drug that can be used to treat their malignancy.”
Inhorn notes that like many patients participating in MCGI, Pierce “understands that there is also an altruistic piece to this. They understand that MCGI and the clinical community are trying to learn and create a larger database of treatment options and to engage and educate oncologists about how to best use these platforms. I think the day is coming where everyone who has an advanced malignancy will be offered genomic profiling — it’s going to become a standard of care.”
Tumor profiles from MCGI testing, and their best treatment options, are reviewed at Genomic Tumor Board (GTB) sessions, virtual conferences that link clinicians with experts in cancer genomics and clinical trials. Inhorn mentioned to Pierce that there was a tumor board meeting coming up, and Pierce said, “I’d like to participate. I’d like to be in the room when they’re discussing my case.”
Dr. Jens Rueter, chief medical officer at JAX, says that Pierce’s participation in the tumor board “worked out really well. Beforehand I was a little bit nervous about it because I didn’t really know what to expect. He’s an unusual individual because he embraces new technologies, and that’s coupled with really wanting to impact his treatment plan dramatically.”
Rueter says GTBs are an important component of MCGI, as they often provide the most significant input to clinicians with respect to applying the genomic information in their patients’ care plan. Typically, four cases are discussed during each one‑hour meeting. A brief case presentation by the treating oncologist is followed by a presentation of the genomic case information. Then, external advisors specializing in precision oncology give their interpretation of the case and provide significant guidance to the oncologist and the medical team. In this case Pierce himself was also able to query the experts.
Genomic testing identified two targetable mutations in Pierce’s cancer. He is now on his second targeted therapy (erlotinib) after four months on the IDH2 inhibitor enasidenib.
According to Rueter, most cancer patients fall into one of two categories. “The first are comfortable doing what their doctor says, maybe asking questions or even questioning some decisions, but basically trusting the doctor. And the second tend to shop around for doctors until they find the one who tells them what they want to hear.” Pierce is in a rare third category, Rueter says. “He just wants to know everything that could possibly be done to address his cancer.”
Pierce is continuing to actively advocate for his health. “I think cancer and oncology clinicians today don’t talk about cures; they talk about control. And as far as I’m concerned, control isn’t really good enough for what I’m looking for. I am looking for a cure.”
A model for personalized cancer care in a rural setting
MCGI is a statewide collaboration of JAX scientists and community oncologists that brings innovative cancer genomic testing, education and drug access infrastructure to Maine. Every oncology practice in the state is a partner in the program. Initially driven by the need for greater availability of cancer genomic testing in Maine, MCGI has become a model for community precision oncology, or personalized cancer care, in a rural setting. Precision oncology uses analysis of a patient’s normal genetics and the specific mutations found in his or her tumors to guide more targeted treatments.
“Over the last four years, we’ve made great progress with precision medicine in Maine, especially in rural areas of the state,” said Rueter. “In the first phase of the initiative, we provided genomic tests to over 1,600 cancer patients, affecting patient lives from Caribou to Kittery. Over the next five years, we will focus our efforts on helping patients navigate the steps of entering genome-informed clinical trials and of accessing targeted therapies as part of their routine medical care.”
The initiative also plans to expand its reach to other areas of the northeast United States beyond Maine.
4. Harnessing our immune systems to attack cancer
Our ability to understand and manipulate cancer cells — as well as suppress and kill them — will continue to accelerate over the coming years, so we may be poised at the brink of an important leap forward for effective cancer treatments.
Cancer immunotherapy has become a hot medical topic over the last decade. While much remains to be learned, there have been startling early successes, and there is exciting potential for many more in the years ahead. Like in so many medical stories, however, it took many years of research to figure out how to help our own immune systems detect and fight tumors. And the work began with a discovery in a mouse.
Removing checkpoints to treat cancer
In 1996, the journal Science published a paper with an interesting finding. In mice, blocking a protein that acts as an immune “checkpoint” resulted in the elimination of cancer cells, and even established tumors, by the immune system. The protein, cytotoxic T-lymphocyte-associated protein 4 (CTLA4), normally acts to suppress the immune system. That may sound strange, but checkpoint proteins such as CTLA4 are important parts of the vital system of checks and balances needed to prevent overactivation and toxic immune responses.
Unfortunately, cancer cells use these checkpoint proteins to evade attack, flying under the immune surveillance radar to grow and thrive. Researchers strove to learn how to put these cells back on the radar again, whether through targeting a protein unique to the cancer cells themselves or by tweaking immune system function. The finding in the Science paper suggested that a new form of therapy could perhaps be developed by removing the checkpoint “brakes” on the immune response in human patients.
Over the past 24 years, it has become clear that cancer immunotherapy is a powerful new option for oncologists and many of their patients. The effects of the new therapies, most of which are known as immune checkpoint blockades, are inconsistent, but when they work, the results can be surprisingly good. As a result, the Science paper’s senior author, James Allison, has become renowned as a pioneer in the immunotherapy field, the subject of acclaimed documentary film “Breakthrough” and was awarded a Nobel Prize in 2018.
Finding a better checkpoint
Clinical trials for antibodies that bind to CTLA4 began in 2000, and by 2010, the first U.S. Food and Drug Administration-approved treatment ipilimumab (marketed as Yervoy) emerged. Unfortunately, the overall survival benefit was, on average, poor. There were also incidences of severe immune toxicity, when the immune system became overactive and attacked normal tissues as well as tumor cells. In the meantime, another checkpoint protein, programmed cell death protein 1 (PD1), came to the fore.
First discovered in mice in 1992, PD1’s biological mechanism attracted attention over the following decade. CTLA4 inhibits immune cell activation early, in the lymph system, so blocking its function can lead to large-scale, systemic immune activity. PD1, on the other hand, inhibits activity in the peripheral tissues, including tumor microenvironments.
Without it the immune activation is more limited and more focused on the tumor area itself. Much of the preclinical focus therefore shifted to PD1 inhibition, and in 2014 the anti-PD1 antibody pembrolizumab (Keytruda) received FDA approval.
Pembrolizumab has been used to treat cancers that previously had no effective therapies, including metastatic cancer and inoperable tumors. But the majority of patients don’t respond, and severe inflammation is an uncommon but potentially dangerous side effect. So why is there such variability in patient response, how can the percentages be improved and how can side effects be minimized? Research is ongoing into these and many other questions as scientists and doctors seek to bring more precision and efficacy to the cancer immunotherapy field.
Returning to the lab to improve therapies
So Palucka made a bold move and traded her white doctor’s coat for a lab coat so she could explore how our immune system might be mobilized to fight cancer. She didn’t let go of her passions, however. Her commitment and care for patients remain as deep as they were decades ago.
Although there are approved immunotherapies already in regular clinical use, many researchers are taking a step back to figure out how to improve them. Not surprisingly, modeling the intricate interplay and signaling between the immune system components themselves and learning how they interact with cancer cells is a key part of JAX’s cancer immunotherapy research program. Leading the effort is Dr. Karolina Palucka, a former clinician who transitioned to research so that she could help improve patient prognoses. And while Palucka is a human immunologist, she is using mice as the foundation for her work to identify how to harness the power of the immune system to prevent and treat cancer.
Experimental mouse models that recreate human immune response mechanisms have become an important resource for immunology research. However, the first generation of these mice didn’t capture the full repertoire of immune cell types and functions, including macrophages and natural killer cells that play critical roles in cancer initiation and immune evasion. Palucka is therefore working to develop mice with an even more complete human cell population to better understand their exact roles in cancer cell viability, growth and potential metastatic spread.
In addition, Palucka is pursuing a therapeutic strategy based on the concept that T cells, the immune cells that are able to reject tumors, can function as anti-cancer drugs when properly armed for the purpose. It’s possible that anti-cancer reactive T-cell populations can be expanded via cancer vaccines, with the potential to greatly enhance the efficacy of current immunotherapies. With careful manipulation, it may soon be possible to safely and reliably tip the balance of T-cell function in favor of its cancer-destroying abilities. It’s an exciting thought, and one that will drive the cancer immunotherapy field even further forward.
5. Taking aim at breast cancer
Sophisticated genomic studies are revealing the mechanisms of tumor formation, pointing the way to new targeted treatment approaches.
When a woman receives a diagnosis of breast cancer, how will it affect her ability to continue her career, take care of her children, celebrate her 50th wedding anniversary? The answer will be as singular as the woman herself.
That’s because her cancer has a distinctive genetic profile. Researchers have discovered dozens of genetic variants associated with breast cancers, and are racing to develop targeted treatments that disarm the destructive power of cancer genes.
New breast cancer cases outnumber all other kinds of cancer for women. For 2017, the latest year for which statistics are available, the U.S. Centers for Disease Control and Prevention reported 250, 520 new cases a year, more than twice as many as the next-most common cancer, lung and bronchus. That year 42,000 American women died of breast cancer.
But those tragic statistics actually represent a significant improvement in breast cancer survival rates. The past two decades have seen a drop in incidence of ductal carcinoma in situ (DCIS), the type of breast cancer most likely to develop into invasive cancer. The American Cancer Society credits improved detection, and fewer women taking hormone therapy for postmenopausal symptoms, for the reduction. And mortality decreased 40% between 1975 and 2017, thanks to better treatments as well as earlier detection.
In fact, says Dr. Edison Liu, breast cancer researcher and president and CEO of The Jackson Laboratory, “If current trends continue, theoretically there would be no breast cancer mortalities by 2045. And though this remains aspirational and may not be able to be achieved, the direction is clear: We are winning.”
JAX breast cancer researcher Edison Liu. JAX photo by Tiffany Laufer.
Creative innovation in treating breast cancers
What were the medical breakthroughs behind the improvement in prognosis for women with breast cancer? Liu credits “decades of creative innovation,” with each generation of scientists building on findings of the previous generation.
An important early insight was that cancer is not a single disease with a single cause, and that the location of a tumor has less bearing on disease progression or mortality than its mechanisms. Better profiling of breast tumors was the first step in developing more targeted and effective treatments.
The next big cancer breakthroughs, in the 1960s, were a combination of chemotherapy (administering multiple drugs simultaneously) and adjuvant therapy, chemotherapy following surgical removal of a tumor, to eliminate any pockets of residual disease. Liu notes that adjuvant therapy was actually pioneered in breast cancer, by researchers working in the Fondazione IRCCS Istituto Nazionale dei Tumori in Milan, Italy.
Before adjuvant therapy, Liu says, “The standard protocol for breast cancer was draconian surgery followed by draconian radiation.” And while a small number of cases still call for such drastic treatment, “Adjuvant therapy eliminated the terrible side effects of radical mastectomy followed by disfiguring radiation that actually hurt the patient’s health.”
Hormone therapy joined the oncologist’s toolkit in the 1970s. The hormones estrogen and progesterone promote the growth of some breast cancers. The cells of these hormone-dependent breast cancers contain proteins called hormone receptors that become activated when hormones bind to them, causing changes in certain genes and stimulating cell growth. This therapy to block the body’s hormone production is aimed at slowing or stopping the growth of hormone-sensitive tumors.
In 1994 and 1995, researchers identified genetic mutations in genes, designated BRCA1 and BRCA2, that turned out to be the most common cause of hereditary breast cancer. In normal cells, these genes help make proteins that repair damaged DNA, but mutated versions can lead to abnormal cell growth and cancer. Since then close to a dozen other genes have been identified that are associated with elevated risk for breast cancer.
In 1998, the FDA approved trastuzumab, better known under the brand name Herceptin, to treat breast cancers that are HER2-receptor positive. A gene called HER2 makes HER2 proteins, receptors on breast cells that normally control how a healthy breast cell grows, divides and repairs itself. In about 25% of breast cancers, the HER2 gene goes into production overdrive, spurring uncontrolled growth and division of breast cells. Trastuzamab works by binding to the HER2 receptor and slowing down cell duplication.
The impact of fundamental science on breast cancer outcome is clear, Liu says, “but equally important is the contribution of early diagnosis through mammographic screening. Catching cancer before it metastasizes saves lives as does adherence to optimal clinical procedures in the delivery of care.” Now, he adds, with genetic screening to identify individuals at high risk for breast cancer, targeted and intensive preventive measures can further reduce cancer mortality.
“All these may sound incremental,” Liu comments, “but you add them all up, it becomes akin to building a dam, brick by brick. And pretty soon you’ve got a pretty effective dam.” Now, he says, the biggest challenge is improving the survival of patients with triple-negative breast cancer — those cancers that are not estrogen-receptor positive, progesterone-receptor positive or HER2 positive.
JAX breast cancer researcher Francesca Menghi. JAX photo by Tiffany Laufer.
New strategies for tackling triple-negative breast cancers
Triple-negative breast cancers (TNBCs) account for about 15% of all breast cancers, and according to Liu, “is today the worst breast cancer to have: the fastest‑growing, the most metastatic.” A study of 50,000 women with breast cancer showed five-year survival rates for patients with a TNBC diagnosis at 77%, compared to 93% for women with other types of breast cancer.
Liu and his lab focus on TNBC. In 2016 the Liu lab published their discovery of a distinct genomic configuration found in about half of all triple-negative breast, ovarian and endometrial cancers, which they dubbed the tandem duplicator phenotype (TDP). Since then the lab has published many additional findings on how mutations in TP53 and BRCA1 genes give rise to the TDP.
The TDP is the result of mutations that cause faulty DNA replication during cell division. Duplications of short stretches of copied DNA are inserted in the genome next to the segments from which they were copied. These tandem duplication sequences disrupt genes at and near their insertion points and double the production of genes that happen to be copied, uninterrupted, in the middle. The Liu lab is now undertaking a multi-pronged investigation of the TDP.
“We want to understand how the TDP evolves over time,” says Francesca Menghi, associate research scientist in the Liu lab. “How do all of these genomic variations occur? To do this we deploy a combination of computational analyses, studies in new mouse models of breast cancer, and experiments using human cancer cell lines that are either proficient or deficient for BRCA1 activity.”
Splicing factor defects and breast cancer
Another category of genetic error may trigger some breast cancers as well as many other diseases. A JAX research team led by Assistant Professor Olga Anczuków studies splicing factors, which control the version of a given gene that is expressed, with the goal of developing molecules to correct defects in splicing factors.
In the highly simplified, so-called central dogma of biology, the genetic instructions written in DNA are copied (transcribed) into messenger RNA (mRNA), forming individual “machines” that each build one or more proteins — large, complex molecules that play critical roles in the body. But there are additional mechanisms that greatly increase the potential variety of proteins.
Following transcription, a process called alternative RNA splicing enables the creation of different spliced mRNA versions that, in turn, can produce proteins with different functions. “Splicing is like the editing process in filmmaking,” Anczuków says. “Just as inserting or deleting a scene in a movie can change the movie’s meaning, RNA splicing can deliver an altered genetic message.”
Not all splices improve the final product, be it movie or protein. Errors in the splicing process have been implicated in many diseases, including cancer. Anczuków’s lab has shown that a splicing factor called TRA2B seemed particularly enriched in TNBC, and now they are focusing on DNA segments known as poison-exons, which play a critical role in maintaining a tight regulation of splicing-factor levels, which is necessary for normal cell functions.
Researchers at JAX and UConn Health have innovated a process to analyze patient tumors, and identified more than 3,000 splicing events that are specific to breast tumors. The results, note the researchers, provide a rich resource of potential therapeutic targets.
Where are we now?
At her Connecticut-based laboratory, Menghi reflects on how research in breast cancers, including TNBC, will ultimately translate into more targeted and effective treatments.
“Every time I talk with my family or friends that don’t have a scientific background,” she says, “there’s one question they always ask me: Are we going to cure cancer? And it’s just so frustrating because in the short term, the individual discoveries are always really small and incremental.”
To answer the question, she says she explains that the rise of sequencing technology over the past decade has enabled an entirely new approach to cancer research and treatment. “Genome sequencing will open up so much in terms of our ability to understand what drives tumor initiation, and as a consequence, our ability to target it, prevent it, diagnose it early, monitor it better, diagnose relapses better, stratify patients and find novel treatments.
“I think that this is a time of progress, and I’m really confident about it,” Menghi says.
Liu adds, “The most gratifying aspect of this is not only that science has advanced so dramatically, but that all elements of the scientific community are coordinating their efforts into providing cures. I have been working in the field of breast cancer for 30 years, and I have seen how basic, clinical and epidemiological sciences, when all work together, have a direct impact on patient lives.”
The rate of advancement is speeding up, Liu says. “So, perhaps 2045 as the year when breast cancer mortality ceases is not such a pipe dream.”